A narrative review of interventional oncology approaches to treating pancreatic cancer
Introduction
Rationale
This narrative review will go over ablative modalities in the treatment of pancreatic cancer, while specifically focusing on percutaneous ablation with irreversible electroporation (IRE), and future applications of ablative techniques. This narrative provides an in-depth review on radiofrequency ablation (RFA), microwave ablation (MWA), IRE and cryoablation techniques in treating pancreatic cancer. The review also aims to focus on its future treatment and summarizes current prospective trials that offer new insight into potential treatment avenues for pancreatic cancer.
Background
In the United States, pancreatic cancer is the 3rd leading cause of cancer-related death and stands as the 7th leading cause of cancer-related mortality worldwide (1). By 2030, pancreatic cancer is anticipated to surpass colorectal cancer and become the 2nd leading cause of cancer-related mortality (2). The disease has a 5-year survival rate of 11% and is characterized by insidious progression with delayed onset of symptoms (1,3). It has been estimated that at diagnosis 80–85% of patients have late-stage, unresectable cancer (4). Risk factors for the disease include smoking, alcohol, diabetes, obesity, chronic pancreatitis, and environmental exposure to toxins (3,5). The anatomical location of the pancreas, lack of robust screening methods and diagnostic tools, aggressive tumor progression, and low response to chemotherapy or radiotherapy all contribute to the low success rate of treatment. The American Cancer Society reports that the estimated risk of developing pancreatic cancer over an individual’s lifetime is around 1 and 64 (6). According to estimations by the American Cancer Society, approximately 62,210 individuals (32,970 men and 29,240 women) are estimated to receive a diagnosis of pancreatic cancer and approximately 49,830 people (25,970 men and 23,860 women) in the United States will die of pancreatic cancer in 2022 (7,8).
Surgical resection is the gold standard and remains the only potentially curative treatment for pancreatic cancer. Still most patients do not qualify for surgical resection. While there is some evidence that neoadjuvant chemo-radiotherapy improves survival (1), adjuvant chemotherapy treatment is considered standard of care treatment (9).
Given the small percentage of patients that qualify for surgery, the vast majority are treated with chemotherapy. The current standard for initiation chemotherapy is FOLFIRINOX (fluorouracil, oxaliplatin, leucovorin, irinotecan) or a combination of gemcitabine and abraxane (10). Additional adjunctive treatments with chemotherapy include radiation techniques and ablation.
Needle-guided ablation is a minimally invasive treatment modality for patients unable to undergo surgical resection. Several ablation modalities have been used to treat pancreatic cancer. Radiofrequency and MWA use heat to ablate tissue. Cryoablation freezes and destroys tissue by changing gas pressure over freeze-thaw cycles. IRE, the newest of these four modalities, uses pulsed current to create permanent pores in cell membranes and instigate cellular apoptosis. Additionally, vascular, ductal, and connective tissue are protected during treatment. IRE also resists the heat sink phenomenon, where vascular blood flow thermoregulates nearby target tissue which prevents complete ablation of tumors proximal to large blood vessels, due to its nonthermal mechanism (11).
This narrative review analyzes ablation options used to treat pancreatic cancer. The review also aims to focus on the IRE ablation modality and its future in the treatment of pancreatic cancer alongside other promising treatment modalities.
Objectives
In this article, we discussed the current treatment modalities for pancreatic cancer, the role of IRE in the treatment of pancreatic cancer, and future advances in IRE. we also compared IRE to other pancreatic cancer treatment modalities. We present this article in accordance with the Narrative Review reporting checklist (available at https://dmr.amegroups.com/article/view/10.21037/dmr-23-14/rc).
Methods
The research strategy used by the authors was based on analyzing and reviewing past research, narrative reviews, and articles on different treatments of pancreatic cancer. The research used emphasized the different ablative modalities used to treat pancreatic cancer, specifically RFA, MWA, cryoablation and IRE. The research used also examined different prospective trials involved in treating pancreatic cancer. We conducted a search of all literature from July 2022–December 2022. The websites used to find the literature included PubMed and Google Scholar. A comprehensive summary of the authors’ search strategy can be found in Table 1.
Table 1
Items | Specification |
---|---|
Date of search | July 2022–December 2022 |
Databases and other sources searched | PubMed, Google Scholar |
Search terms used | Radiofrequency ablation, microwave ablation, irreversible electroporation, cryoablation, pancreatic cancer, chemotherapy, FOLFIRINOX, PELICAN, or PANFIRE |
Timeframe | 05/2000–11/2022 |
Inclusion and exclusion criteria | Inclusion: different treatments of primary pancreatic cancer; exclusion: neuroendocrine tumor of the pancreas |
Selection process | An author conducted the initial literature search, and consensus was obtained through group discussion with all authors |
Discussion
Chemotherapy
Chemotherapy is primarily indicated for treatment of pancreatic cancer as adjuvant therapy with some patients receiving neo-adjuvant therapy to downstage unresectable or borderline resectable pancreatic cancer (BRPC). First-line adjuvant therapy for pancreatic cancer classically consisted of either gemcitabine or fluoropyrimidine, however, FOLFIRINOX is now the most used chemotherapeutic regimen (12). The PRODIGE 24/CANADIAN Cancer Trials group demonstrated superior 5-year outcomes of a modified FOLFIRINOX regimen compared to gemcitabine in an open-label, phase 3 randomized clinical trial. Both medications, modified FOLFIRINOX or gemcitabine, were given over 24 weeks to patients with confirmed pancreatic ductal adenocarcinoma treated with macroscopic resection 3–12 weeks prior to adjuvant chemotherapy. The trial consisted of 77 hospitals in France and Canada and 493 total patients. Adjuvant gemcitabine therapy resulted in a median disease-free survival of 12.8 months, median overall survival (OS) of 35.5 months, and median metastasis-free survival of 17.7 months. Adjuvant modified FOLFIRINOX treatment achieved a significantly improved median disease-free survival of 21.4 months, median OS of 53.5 months, and median metastasis-free survival of 29.4 months. The final 5-year result showed that modified FOLFIRINOX led to longer survival compared to gemcitabine in the adjuvant setting for patients with resected pancreatic ductal adenocarcinoma (13).
The overwhelming success of FOLFIRINOX over its predecessors posed new questions regarding the role of locoregional therapy in pancreatic cancer treatment. Byun et al. investigated whether resection followed by adjuvant FOLFIRINOX resulted in improved survival over FOLFIRINOX alone. The study consisted of 337 patients [67 with BRPC, 135 with locally advanced pancreatic cancer (LAPC), and 135 with metastatic pancreatic cancer] who received FOLFIRINOX-based chemotherapy either alone or as an adjuvant therapy to surgery. Chemotherapy was given over a median of nine cycles with a median duration of 168 days in this study, and eligibility for surgery was primarily determined using computed tomography (CT) scans taken after four to six cycles. In patients determined ineligible for surgery or other locoregional therapy, FOLFIRINOX-based chemotherapy alone resulted in a median survival of 23, 19, and 14 months among patients initially with BRPC, LAPC, and metastatic pancreatic cancer respectively. In patients who received FOLFIRINOX as adjuvant therapy to surgical resection a median survival of 35 and 32 months was achieved amongst those with BRPC and metastatic pancreatic cancer respectively. The study could not predict median survival in those with LAPC who received surgery and adjuvant chemotherapy. Byun et al. concluded that although their clinical outcomes with FOLFIRINOX therapy alone far surpassed reported outcomes of gemcitabine therapy, the addition of surgical resection in treatment offered a clear survival benefit regardless of the clinical stage of the disease (12). This suggests that locoregional therapies continue to have an important role in the treatment of pancreatic cancer in the FOLFIRINOX era.
RFA
RFA, uses alternating current via one or more needle electrodes to ablate tissue. The current agitates water molecules, generating frictional heat within the tissue, with target cells experiencing coagulative necrosis and protein denaturation (14). Despite its widespread availability and low cost, data is limited on RFA’s benefits in pancreatic cancer.
Ruarus et al. (4) reviewed the oncological outcomes of RFA in the treatment of LAPC. The review examined six studies, two retrospective and four prospective. The retrospective studies included 80 total patients, and the prospective studies included 270 total patients. Open laparotomy was used in five studies, while a percutaneous approach was used in one study. The longest reported median follow-up time was 15 months by Paiella et al. (14). An OS ranging from 19.0 to 25.6 months was reported in four of the six studies. A study in the review, Cantore et al. (3), showed that the median OS was significantly higher (25.6 months) for the patients who underwent RFA as a second line treatment after chemotherapy, compared to first line RFA (14.7 months). The review reported morbidity and 30-day mortality rates within the range of 0–28% and 0–3% respectively.
More recently, a phase II study analyzed the safety of RFA in patients with LAPC. The aim of the study was to analyze safety of RFA, measured as the number of patients with major complications within 30 days of the procedure or during the initial admission. Fegrachi et al. performed RFA on 17 patients with LAPC and measured major complications (Clavien-Dindo ≥3). RFA related complications included pancreatitis, pancreatic fistula, thermal damage to portomesenteric vessels and duodenal perforation. Nine patients experienced major complications (Clavien-Dindo grade ≥3). Eight patients experienced delayed gastric emptying (DGE) requiring an endoscopic tube, with DGE being the only major complication for four patients. Five patients experienced a major complication other than DGE, and one patient died 57 days after RFA due to a hepaticojejunostomy leakage with intra-abdominal abscesses, cholangiosepsis with liver abscesses plus respiratory failure. One patient developed a pseudoaneurysm, defined as a complication related to RFA. The authors stated that morbidity after RFA of the pancreas is mostly due to DGE, which mainly happened after surgical gastrojejunostomy. This study allowed for the effects of RFA alone to be investigated, and, overall, the authors determined that RFA is considered safe when safety measures are followed for patients with LAPC (15).
A recently published study protocol on a randomized controlled trial (RTC) compared the OS of patients with LAPC that were treated with both RFA and chemotherapy versus chemotherapy alone. The PELICAN (Pancreatic Locally Advanced Unresectable Cancer Ablation) trial screens patients for eligibility after 2 months of treatment with either four cycles of FOLFIRINOX or two cycles of nab-paclitaxel/gemcitabine. The measured outcomes include OS, progression-free survival (PFS), RECIST response, pain, quality of life (QOL), tumor markers, costs, immunomodulatory effects of RFA, and toxicity of chemotherapy for the RFA plus chemotherapy group versus the chemotherapy alone group (16). The study has been estimated to finish recruiting by December of 2021.
MWA
MWA employs electromagnetic pulses to generate frictional heat and coagulative necrosis in the target zone by drastically moving dipoles. This modality produces larger ablation zones, generates higher lethal temperatures in a shorter time period, and demonstrates an improved convection profile over RFA (17). Due to the delicate structure of the pancreas and its anatomical location nearby to other critical organs such as the duodenum, common bile duct, splenic vessels, portal vein and abdominal aorta, ablative techniques must be used with caution and extreme precision. MWA has several advantages over RFA such as more predictable ablation volumes, more precise margins, shorter time to reaching lethal temperatures, improved resistance to the heat-sink effect, and greater efficiency in lesions with cystic components (18).
Carrafiello et al. (19) conducted a review to assess the safety and efficacy of percutaneous MWA for treating locally advanced, nonresectable, nonmetastatic cancer in the head of the pancreas in 10 patients. Five patients were treated percutaneously and five during laparotomy with MWA using a 10-minute ablation time. The technical success rate was 100% and one major complication was observed. One patient developed a pseudoaneurysm of the gastroduodenal artery. This complication was treated via an endovascular approach. All patients experienced an improved QOL after the procedure, but there was tendency for patients to return to pre-operative levels within 9 months. According to the Response Evaluation Criteria in Solid Tumours (RECIST) criteria, 9-month local tumor progression was 37.5% (3,8) and 1-year local tumor progression was 62.5% (5,8).
Vogl et al. did a retrospective evaluation of 22 MWA sessions in 20 patients with primary pancreatic cancer between August and March 2017 (20). All patients underwent CT-guided percutaneous MWA using the same high-frequency (2.45 GHz) MWA device. Seventeen tumors were located in the head of the pancreas and five in the tail. There were no major complications observed and technical success was seen in 100% of the patients. The post-ablation diameter was 3.4 cm and the mean ablation volume was 7.8 cm (20). Only 10 of the 22 tumors had available 3-month follow-up imaging. Only one of these 10 tumors demonstrated local tumor progression.
Lygidakis et al. retrospectively studied 15 patients treated with MWA during laparotomy (21). Partial necrosis was achieved in all the participants. There were no reports of major morbidity or mortality related to the procedure and six patients had minor complications. The patient that survived the longest was monitored with a 22-month follow-up.
Data on MWA of pancreatic adenocarcinoma is sparse with high variation in reported oncological outcomes. Most studies discuss tumor progression but do not examine OS. More data on survival following MWA is needed to assess the value of this treatment modality.
Cryoablation
Cryoablation freezes tissue to induce cell death. Probes are placed into target tissue and argon is forced through the narrow opening at the distal tip of the probe under high pressure. The gas rapidly expands to atmospheric pressure and the surrounding tissue is cooled by the Joule-Thompson effect (temperature reduction with rapid gas expansion). The gas is then vented out, leading to warming of the probe and thawing of tissue. Cooling and thawing tissue induces changes in cell osmolarity, organelle and plasma membrane disruption, ischemia, and apoptosis which kill cells. The ablation field can be estimated in real-time within the space of the larger “ice-ball”, visible via ultrasound or CT, generated during cryoablation (22). The cooling of tissue has an anesthetic effect, which makes this modality less painful than heat-based ablation modalities. Cryoablation does tend to produce stronger inflammatory responses than other ablation modalities (23). This can manifest as a systemic inflammatory response known as cryo-shock, which is characterized by multiorgan failure and coagulopathy (24).
Niu et al. (25) conducted a retrospective study assessing the feasibility of percutaneous cryoablation for pancreatic tumors. Thirty-two patients diagnosed with stage II/III/IV pancreatic cancer and tumors measuring 2–11 cm were treated. Thirteen tumors were located in the pancreatic head and 19 tumors were located in the pancreatic body and tail. Forty-nine procedures were performed, and outcomes were analyzed 1–3 months afterwards. In a single session of cryoablation, 15 tumors were completely ablated, and all were smaller than 5 cm. With a second cycle of ablation, all other tumors were controlled with or without brachytherapy. One to three months after the first ablation session, residual enhancing nodules were detected at the rim of 13 lesions. Eleven of the nodules had no signs of progression in situ during follow-up. The remainder of the nodules were controlled with brachytherapy. Partial response was seen in nine patients, stable disease was seen in 21 patients, and progressive disease was seen in two patients. Clinical benefit response was seen to be 84.4%. A greater than 50% reduction in pain scores was seen in 27 patients. Twenty-two patients reported a greater than 50% reduction in analgesic consumption after ablation. Furthermore, the rate of clinical benefit response showed no significant differentiation between large and small tumors. The mean survival time was 15.9 months. The 6-month OS was 82.8%, the 12-month OS was 54.7%, and the 24-month OS was 27.3%.
A more recent study by Wu et al. (6) affirmed these findings. Laparoscopic cryoablation of LAPC in 10 patients (seven of whom received complete ablation of tumors visible on imaging) reduced mean CA19-9 levels from 347.5 to 190.4 U/mL at one week with sustained reduction observed at 3 months and CT imaging suggestive of tumor necrosis in cryo-ablated areas. Mean pain scores declined from an average of 6.9 prior to operation to 1.3 and 2.0 at 1 and 3 weeks respectively. No major complications were observed (6).
Although data is limited, the analgesic effect and lack of serious adverse events associated with cryoablation suggest that it could serve as an effective palliative treatment.
IRE
IRE is a non-thermal, ablation modality that induces cell destruction and injury by creating nanopores in the cellular phospholipid bilayer. This is achieved with rapid pulses of high-voltage, low-energy DC current. The procedure can be performed percutaneously, laparoscopically, or via an open approach. Two or more electrodes (up to a maximum of six) are placed in or around the tumor. The electric fields from IRE disrupt cellular homeostasis and induce cell death (6). IRE is primarily a non-thermal ablation modality, though some heat-associated necrosis has been observed at higher voltages. This primarily non-thermal mechanism allows IRE to resist the heat-sink phenomenon in which high blood flow in vasculature near ablation targets thermoregulate tissue and mitigate ablative effects in tissue, that plagues thermal ablation. IRE spares vascular, ductal, and connective tissues within its ablation zone and can produce sharper ablation borders than other ablative modalities (7).
Retrospective studies
Charpentier et al. first studied IRE in swine models (8). Four female swine models were utilized to study IRE on the pancreas. Two monopolar probes, spaced 9–15 mm apart, were used. All animals survived at 2 hours (n=1), 2 days (n=1), and 14 days (n=2). The study had no treatment related complications to report. IRE was determined to be a safe and viable treatment modality method for pancreatic tissue ablation. A study using mouse models (26) for IRE therapy observed alterations to the tumor microstructure 30 minutes after ablation. Early passage PANC-1 cells were harvested and implanted into both the left and right flanks of 14 mice. The tumors grew to around 8 mm in a 3–4-week timeframe before treatment. Mice were randomly assigned into two groups. In the first group (n=8), IRE ablation was done on the right sided tumors, while left flank tumors were untreated. Both left and right sided lesions were treated in the second group (n=6) with IRE ablation. Diffusion-weighted magnetic resonance imaging (MRI) apparent diffusion coefficient measurements were used to compare immunochemistry markers before and after IRE. When comparing the two groups of mice, the apoptosis index measurements were found to be significantly higher in IRE-treated tumors than in control groups. After 30 minutes of IRE ablation, investigators observed tissue alterations consisting of morphological and structural changes with significantly increased apoptosis biomarkers (26).
IRE of pancreatic cancer in humans was first performed via an open approach before the development of the image-guided percutaneous technique. The percutaneous technique was first described by Narayanan et al. in 14 patients over 15 ablations for tumors of median size 3.3 cm (range, 2.5–7 cm). Three patients presented with metastatic disease and eleven presented with LAPC. Chemotherapy was given to all participants prior to the intervention and 11 were given radiation therapy. An OS of 23.3 months was observed from the time of diagnosis and 18 months from the IRE treatment. Two participants were down staged and underwent margin-negative resections at 4 and 5 months respectively after IRE treatment. One complete pathologic response was reported (27).
Narayanan et al. (28) later conducted a retrospective analysis of 50 patients treated with percutaneous CT-guided IRE for LAPC that was not amendable to surgery. In the study, 30-day mortality was 0% and no treatment related deaths were reported. Serious (grade 3 or 4) adverse events were observed in 20% of participants. Median OS was 27 and 14.2 months from the time of diagnosis and time of IRE respectively. Lesions smaller than 3 cm exhibited a significantly extended OS compared to larger tumors. Martin et al. later conducted a study of IRE performed with an open surgical approach where a cohort of 200 participants with unresectable lesions were treated (29). Median OS of 23.3 and 18 months were achieved from the time of diagnosis and from the time of IRE respectively. Both studies reported 0% mortality rate at 30 days and no treatment related deaths. There was an 18% adverse event rate with complications graded as serious (grade 3 or 4) events. Figure 1 shows a 67-year-old female with stage 3 LAPC centered in the pancreatic body encasing the celiac trunk, superior mesenteric artery (SMA), superior mesenteric vein (SMV) and portal vein confluence treated with percutaneous IRE.
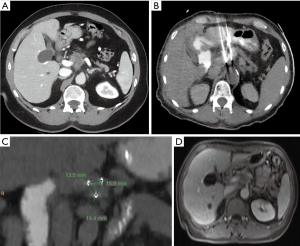
A large retrospective study of 75 participants diagnosed with LAPC received percutaneous IRE post chemotherapy reported a 27-month median OS and a 15-month PFS (30). The procedure down-staged LAPC in four non-surgical candidates for surgical resection, with R0 resections achieved in 3 cases (31). Mortality up to 30 days post treatment was 0% and the total amount of adverse events was 25%. The findings of this study indicate that the use of percutaneous IRE for LAPC was deemed safe when combined with standard of care chemotherapy. Furthermore, this treatment method holds the potential for enhancing OS rates. Shen et al. conducted a retrospective study at a single institution exploring the safety and efficacy of IRE against LAPC for 210 patients. Post-operative complications in this study consisted of DGE, intra-abdominal hemorrhage, and intra-abdominal infection. Patients in this retrospective study were split into three groups: LAPC at the pancreatic head or neck treated with IRE, palliative surgery, and pancreaticoduodenectomy with vascular resection. The vascular resection group was used as a positive control while the palliative surgery group was used as a negative control. Compared to the vascular resection group, the IRE group experienced lower incidences of pancreatic fistulas and intra-abdominal infections, but no significant difference in the rates of pancreatitis, re-operation, or death (32). A retrospective review by He and Li also noted that complications following IRE were similar to those of other modes of ablation. These complications included sepsis, gastric leak, duodenal edema, portal thrombosis, pseudoaneurysm bleeding, intra-abdominal abscess, biliary obstruction, duodenal bleeding, portal vein thrombosis, and strictures (30).
Comparing safety, survival, and tumor recurrence following percutaneous and open-surgery approaches, Sugimoto et al. retrospectively reviewed outcomes of eight patients with histologically proven LAPC ≤5 cm treated via IRE (33). Four patients underwent percutaneous IRE under ultrasound guidance, and four patients had the procedure done by an open approach. The approach made no difference in the rates of serious complications, and 90-day mortality was at 0%. A median OS of 24 and 17.5 months from the time of diagnosis and treatment respectively were observed, paralleling outcomes reported by Narayanan et al. and Martin et al. These studies showed the potential feasibility of a percutaneous approach, as well as an open approach, of IRE for treating LAPC.
He et al. (34) compared IRE to RFA after induction chemotherapy for patients with LAPC. Fifty-eight patients diagnosed with LAPC treated with RFA or IRE after induction chemotherapy treatment were retrospectively reviewed. The survival outcomes after IRE (n=36) and RFA (n=18) were compared. After propensity score matching (PSM) analysis, IRE showed increased survival benefit: 2-year OS, 53.5% vs. 27.0% and 2-year PFS, 28.4% vs. 6.4% for IRE vs. RFA respectively. For tumors smaller than 4 cm, IRE could be explored as an alternative treatment modality to RFA with the potential of having promising results for positive outcomes.
Published prospective trials
Data obtained from the previously described IRE studies paved the way for prospective trials. PANFIRE 1 (Percutaneous Irreversible Electroporation in Locally Advanced and Recurrent Pancreatic Cancer) investigated the feasibility of percutaneous IRE for LAPC by evaluating QOL, pain perception, efficacy, event-free survival, and OS (35). Twenty-five patients with histologically proven LAPC 5 cm or smaller underwent percutaneous CT-guided IRE [median tumor size was 4.0 cm (range, 3.3–5.0 cm) in diameter]. With a median follow-up period of 12 months, there was a reported 8 months of event free survival. After IRE treatment, there was a median time to local progression of 12 months. Median OS was reported as 11 and 17 months from IRE and time of diagnosis respectively. Twelve grade I and II complications were observed and 11 grade III and IV complications were reported in 10 patients. At 90 days post IRE, there were no deaths reported.
The PANFIRE II study followed soon after and was a multicenter, prospective, single-arm trial of patients with locally recurrent pancreatic cancer or LAPC (36). Fifty participants, 25 men and 25 women with a median age of 61 years, were included. Forty patients were diagnosed with LAPC and 10 patients had local recurrence after pancreatic tumor resection. Median OS was 17 months from diagnosis of LAPC [95% confidence interval (CI): 15–19] and 10 months from IRE (95% CI: 8–11). In the LAPC group, 18 participants received no therapy or gemcitabine-based induction chemotherapy, while 22 received FOLFIRINOX. Patients that received FOLFIRINOX, compared to patients that received gemcitabine or no chemotherapy before IRE, did not show improved survival [hazard ratio (HR) =1.1; P=0.70]. This study showed that IRE could serve as a key factor in extending the OS in patients with pancreatic cancer.
A study by Liu et al., published in 2019 (37), prospectively enrolled 54 patients with stage III/IV pancreatic cancer that underwent IRE either with or without chemotherapy to analyze PFS, OS, and safety based on the occurrence of adverse events. Twenty-six patients had stage IV pancreatic cancer, and 28 patients had stage III. Thirty-one patients received IRE with chemotherapy (gemcitabine 26 patients; FOLFIRINOX 5 patients) and 23 only received IRE. No deaths relating to the IRE procedure were reported. Common adverse events reported were ascites (n=15), pleural effusion (n=14), fever (n=19), and abdominal pain (n=6). Four major complications were observed. Three patients developed duodenal hemorrhage at 11, 15, and 21 days respectively after IRE and one patient developed portal vein thrombosis 9 days after IRE (this patient was in a hypercoagulative state prior to IRE and was administered nadroparin for anticoagulation). Patients with stage III disease had a median follow-up of 18.8 months with a range of 9.6–28.7 months while patients with stage IV disease had a median follow-up of 13.3 months with a range of 3.7–23.1 months. Patients with stage III disease had a median OS of 16.2 months from diagnosis and 20.3 months for the IRE and IRE + chemotherapy groups respectively. In the patients with stage IV disease, the median OS from diagnosis was 11.6 and 13.6 months in the IRE and IRE + chemotherapy groups, respectively.
van Veldhuisen et al. conducted a post hoc comparison on data from a prospective IRE-FOLFIRINOX cohort and a retrospective FOLFIRINOX-only cohort. They found an improved median OS of 17.0 vs. 12.4 months and time to progression of 14.2 vs. 5.2 months in the IRE-FOLFIRINOX group vs. the FOLFIRINOX only group. All 52 patients (IRE-FOLFIRINOX group: 30, FOLFIRINOX only group: 22) in this comparison were treated with a minimum of three cycles of FOLFIRINOX for LAPC. This study concluded that combination of IRE and FOLFIRINOX may be a feasible treatment modality in the future for treatment of pancreatic cancer (38).
Ongoing prospective trials
PANFIRE III assesses the safety of IRE combined with immunotherapeutics such as IMO-2125 (toll-like receptor 9 ligand) and/or nivolumab in patients with metastatic pancreatic ductal adenocarcinoma (mPDAC) (39). PANFIRE III aims to see if combining IRE with PD-1 checkpoint inhibition (nivolumab) and effective dendritic cell priming through intratumoral injection of IMO-2125 might establish in vivo immunization and durable treatment results in mPDAC patients. This study is a randomized controlled phase I clinical trial where 18 patients with mPDAC pre-treated with chemotherapy will be enrolled in one of three study arms: A (control): nivolumab monotherapy; B: percutaneous IRE of the primary tumor followed by nivolumab; or C: intratumoral injection of IMO-2125 followed by percutaneous IRE of the primary tumor and nivolumab. It is hypothesized that such combinations could allow the immune system to initiate systemic tumor degeneration and protection against further tumor growth and spread. As there is no curative treatment for mPDAC, innovative and novel treatment approaches are the most important next steps. If electro immunotherapy can become an effective anti-tumor response and treatment, it may set the stage for improvement of PDAC’s prognosis.
The CROSSFIRE trial (40), a phase 3, multicenter, RTC, is investigating stereotactic ablative radiotherapy (SABR) versus percutaneous IRE after neoadjuvant FOLFIRINOX in patients with LAPC. The primary outcomes are PFS, local progression-free survival (LPFS), OS, safety/toxicity, QOL, immunomodulation, tumor marker CA19-9, and total direct and indirect costs as secondary outcomes. A total of 138 patients diagnosed with locally advanced pancreatic adenocarcinoma [American Joint Committee on Cancer (AJCC) stage III] aged 18 years and older will be enrolled.
The DIRECT trial is a 2-arm, unblinded, multicenter RTC that will compare IRE with chemotherapy against chemotherapy alone (41). Control arm subjects are treated with the modified FOLFIRINOX regimen alone, while the experimental IRE arm subjects are treated with both the modified FOLFIRINOX regimen and IRE (either open or percutaneous approach). All patients will be initially treated with the modified FOLFIRINOX regimen for at least 3 months. Then the patients will be randomly split into the control or IRE arm after completion of the 3-month modified FOLFIRINOX chemotherapy treatment. Participants must have no evidence of disease progression after completion of the 3-month modified FOLFIRINOX chemotherapy treatment in order to participate in the randomized control trial (RCT).
Future of IRE
The immunomodulatory effect of IRE is an important area of research. The immunosuppressive environment of the pancreatic stroma leads to limited efficacy of immunotherapy against pancreatic cancers. Pancreatic ductal carcinoma is characterized by a stroma microenvironment that is highly fibrotic. This environment can exclude T cells from the tumor cells (42). The pancreatic stroma environment can also inhibit the activity of T cells. The stellate cells within the stroma of the pancreas interact with cancer cells to evade immune surveillance, modulate cell proliferation, modulate extracellular matrix formation, and help to promote angiogenesis. Zhao et al. (42) conducted a study in the murine model where IRE was used to modulate the tumor-associated stroma of pancreatic ductal adenocarcinoma. IRE, in this model, induced immunogenic cell death, activated dendritic cells, and relieved stroma-induced immunosuppression. With the combination of IRE and anti-programmed cell death protein 1 immune checkpoint blockade, tumor infiltration by CD8+ T cells was promoted and significantly prolonged survival in the model. There was also evidence of a long-term memory immune response. Under CD3 staining, the T cell count was almost doubled in the IRE group at 6-, 12-, and 24-hour mark compared to the cryoablation group. This study showed that IRE has potential to increase the efficacy of the immunosuppression in pancreatic ductal adenocarcinoma.
A study using mice models has also been used to assess the combined effects of IRE and cryoablation on the immune response in pancreatic cancer. When the two modalities were compared, IRE was found to elicit a more significant immune response with infiltration of macrophages and T cells when compared to cryoablation within 24 hours (43). This study showed promise in the ability of IRE to modulate the immune response against pancreatic cancer and its role in immunotherapy.
Imran et al. (44) found a similar effect where IRE treatments in a subcutaneous flank model of pancreatic cancer significantly delayed cancer progression by changing the cancer microenvironment into a pro-inflammatory state. Cytotoxic CD8+ T cells were recruited into the tumor site and increased interferon gamma levels in the serum, showing the potential of IRE as an immunomodulatory treatment modality.
Combining immunotherapeutic approaches with IRE is at the forefront of pancreatic cancer treatment (45,46). Adoptive cell transfer therapy (ACT) is one such modality. ACT, using the infusion of ex vivo expanded or engineered immune cells, aims to generate an immune mediated anti-tumor response in the body. One pancreatic cancer treatment avenue that has been explored is the adoptive transfer of T cells specific for MUC1 (a tumor antigen expressed in invasive ductal carcinomas of the pancreas). MUC1 specific T cells have shown a strong tumor cytotoxicity, allowing the exploration of their use in the treatment of pancreatic cancer. Studies have also shown that therapies involving natural killer (NK) cells are promising in the early host defense against some cancers, including pancreatic cancer.
In a study of 40 patients with metastatic pancreatic cancer, 20 received IRE alone and 20 received an autologous NK cell infusion after undergoing ablative IRE. The lymphocyte counts and the type 1 cytokine levels were significantly higher in the IRE-NK group post-treatment while type 2 cytokines remained unchanged. At the 1- and 2-month mark post treatment, CA19-9 and CA242 expression was lower in the IRE-NK group. The IRE adaptative NK cell transfer therapy was well tolerated in patients and a synergistic effect, as well as an improved anti-tumor response, was noted (47). At the 2-month mark, the IRE-NK group had a smaller maximum tumor diameter. The potential of a synergistic relationship between IRE and immunotherapy can prove to be beneficial for patients with pancreatic cancer.
High-frequency IRE is a new emerging modality that utilizes bipolar square waves of 1–5 µs in rapid bursts for treatment. The benefit of this technique is the elimination of the need for cardiac synchronization and paralytics. The novel aspect of this modality is that it addresses the current limitation of IRE as a treatment modality as it reduces the risk of muscle tetany and cardiac asynchrony. High-frequency IRE can be performed with a single-needle, dual electrode device which will remove the need to place and align multiple electrodes (48).
The above studies provide evidence of the larger role that IRE can play in the treatment of pancreatic cancer and how the evolving treatment modality can lead to tumor ablation and immune system modulation in the treatment of pancreatic tumors.
Conclusions
Pancreatic cancer is a complex and often fatal disease. The silent nature of this disease’s progression leads to most patients presenting with unresectable tumors, leaving a small percentage of patients that can qualify for surgery. Despite the array of treatments present in our modern arsenal, conventional treatment has not improved survival in patients and this cancer continues to present with high mortality rates.
Ablation technologies have continued to evolve starting with RFA and more recently with IRE and so has the quality of the related studies and data. The combination of immunotherapy with these ablation modalities and the potential to stimulate an immune response using some of these technologies offers hope for the future in the fight against pancreatic cancer.
Acknowledgments
Funding: None.
Footnote
Provenance and Peer Review: This article was commissioned by the Guest Editor (Michael D. Chuong) for the series “Novel Therapies for Pancreas Cancer” published in Digestive Medicine Research. The article has undergone external peer review.
Reporting Checklist: The authors have completed the Narrative Review reporting checklist. Available at https://dmr.amegroups.com/article/view/10.21037/dmr-23-14/rc
Peer Review File: Available at https://dmr.amegroups.com/article/view/10.21037/dmr-23-14/prf
Conflicts of Interest: All authors have completed the ICMJE uniform disclosure form (available at https://dmr.amegroups.com/article/view/10.21037/dmr-23-14/coif). The series “Novel Therapies for Pancreas Cancer” was commissioned by the editorial office without any funding or sponsorship. G.N. is a Consultant for Angiodynamcis, Boston Scientific endoscopy, Stryker and Varian Interventional Solutions. G.N. is also on the advisory board for All Vascular. The authors have no other conflicts of interest to declare.
Ethical Statement: The authors are accountable for all aspects of the work in ensuring that questions related to the accuracy or integrity of any part of the work are appropriately investigated and resolved.
Open Access Statement: This is an Open Access article distributed in accordance with the Creative Commons Attribution-NonCommercial-NoDerivs 4.0 International License (CC BY-NC-ND 4.0), which permits the non-commercial replication and distribution of the article with the strict proviso that no changes or edits are made and the original work is properly cited (including links to both the formal publication through the relevant DOI and the license). See: https://creativecommons.org/licenses/by-nc-nd/4.0/.
References
- McGuigan A, Kelly P, Turkington RC, et al. Pancreatic cancer: A review of clinical diagnosis, epidemiology, treatment and outcomes. World J Gastroenterol 2018;24:4846-61. [Crossref] [PubMed]
- Scopelliti F, Pea A, Conigliaro R, et al. Technique, safety, and feasibility of EUS-guided radiofrequency ablation in unresectable pancreatic cancer. Surg Endosc 2018;32:4022-8. [Crossref] [PubMed]
- Cantore M, Girelli R, Mambrini A, et al. Combined modality treatment for patients with locally advanced pancreatic adenocarcinoma. Br J Surg 2012;99:1083-8. [Crossref] [PubMed]
- Ruarus A, Vroomen L, Puijk R, et al. Locally Advanced Pancreatic Cancer: A Review of Local Ablative Therapies. Cancers (Basel) 2018;10:16. [Crossref] [PubMed]
- Faroja M, Ahmed M, Appelbaum L, et al. Irreversible electroporation ablation: is all the damage nonthermal? Radiology 2013;266:462-70. [Crossref] [PubMed]
- Wu Y, Gu Y, Zhang B, et al. Laparoscopic ultrasonography-guided cryoablation of locally advanced pancreatic cancer: a preliminary report. Jpn J Radiol 2022;40:86-93. [Crossref] [PubMed]
- Narayanan G, Bhatia S, Echenique A, et al. Vessel patency post irreversible electroporation. Cardiovasc Intervent Radiol 2014;37:1523-9. [Crossref] [PubMed]
- Charpentier KP, Wolf F, Noble L, et al. Irreversible electroporation of the pancreas in swine: a pilot study. HPB (Oxford) 2010;12:348-51. [Crossref] [PubMed]
- Conroy T, Bachet JB, Ayav A, et al. Current standards and new innovative approaches for treatment of pancreatic cancer. Eur J Cancer 2016;57:10-22. [Crossref] [PubMed]
- Conroy T, Hammel P, Hebbar M, et al. FOLFIRINOX or Gemcitabine as Adjuvant Therapy for Pancreatic Cancer. N Engl J Med 2018;379:2395-406. [Crossref] [PubMed]
- Narayanan G, Daye D, Wilson NM, et al. Ablation in Pancreatic Cancer: Past, Present and Future. Cancers (Basel) 2021;13:2511. [Crossref] [PubMed]
- Byun Y, Han Y, Kang JS, et al. Role of surgical resection in the era of FOLFIRINOX for advanced pancreatic cancer. J Hepatobiliary Pancreat Sci 2019;26:416-25. [Crossref] [PubMed]
- Conroy T, Castan F, Lopez A, et al. Five-Year Outcomes of FOLFIRINOX vs Gemcitabine as Adjuvant Therapy for Pancreatic Cancer: A Randomized Clinical Trial. JAMA Oncol 2022;8:1571-8. [Crossref] [PubMed]
- Paiella S, Salvia R, Ramera M, et al. Local Ablative Strategies for Ductal Pancreatic Cancer (Radiofrequency Ablation, Irreversible Electroporation): A Review. Gastroenterol Res Pract 2016;2016:4508376. [Crossref] [PubMed]
- Fegrachi S, Walma MS, de Vries JJJ, et al. Safety of radiofrequency ablation in patients with locally advanced, unresectable pancreatic cancer: A phase II study. Eur J Surg Oncol 2019;45:2166-72. [Crossref] [PubMed]
- Walma MS, Rombouts SJ, Brada LJH, et al. Radiofrequency ablation and chemotherapy versus chemotherapy alone for locally advanced pancreatic cancer (PELICAN): study protocol for a randomized controlled trial. Trials 2021;22:313. [Crossref] [PubMed]
- Simon CJ, Dupuy DE, Mayo-Smith WW. Microwave ablation: principles and applications. Radiographics 2005;25:S69-83. [Crossref] [PubMed]
- Ierardi AM, Biondetti P, Coppola A, et al. Percutaneous microwave thermosphere ablation of pancreatic tumours. Gland Surg 2018;7:59-66. [Crossref] [PubMed]
- Carrafiello G, Ierardi AM, Fontana F, et al. Microwave ablation of pancreatic head cancer: safety and efficacy. J Vasc Interv Radiol 2013;24:1513-20. [Crossref] [PubMed]
- Vogl TJ, Panahi B, Albrecht MH, et al. Microwave ablation of pancreatic tumors. Minim Invasive Ther Allied Technol 2018;27:33-40. [Crossref] [PubMed]
- Lygidakis NJ, Sharma SK, Papastratis P, et al. Microwave ablation in locally advanced pancreatic carcinoma—a new look. Hepatogastroenterology 2007;54:1305-10. [PubMed]
- Erinjeri JP, Clark TW. Cryoablation: mechanism of action and devices. J Vasc Interv Radiol 2010;21:S187-91. [Crossref] [PubMed]
- Chapman WC, Debelak JP, Wright Pinson C, et al. Hepatic cryoablation, but not radiofrequency ablation, results in lung inflammation. Ann Surg 2000;231:752-61. [Crossref] [PubMed]
- Ní Eochagáin A. Cryoshock following cryoablation for hepatocellular carcinoma. J Clin Anesth 2022;77:110641. [Crossref] [PubMed]
- Niu L, He L, Zhou L, et al. Percutaneous ultrasonography and computed tomography guided pancreatic cryoablation: feasibility and safety assessment. Cryobiology 2012;65:301-7. [Crossref] [PubMed]
- Zhang Z, Li W, Procissi D, et al. Rapid dramatic alterations to the tumor microstructure in pancreatic cancer following irreversible electroporation ablation. Nanomedicine (Lond) 2014;9:1181-92. [Crossref] [PubMed]
- Narayanan G, Hosein PJ, Arora G, et al. Percutaneous irreversible electroporation for downstaging and control of unresectable pancreatic adenocarcinoma. J Vasc Interv Radiol 2012;23:1613-21. [Crossref] [PubMed]
- Narayanan G, Hosein PJ, Beulaygue IC, et al. Percutaneous Image-Guided Irreversible Electroporation for the Treatment of Unresectable, Locally Advanced Pancreatic Adenocarcinoma. J Vasc Interv Radiol 2017;28:342-8. [Crossref] [PubMed]
- Martin RC 2nd, Kwon D, Chalikonda S, et al. Treatment of 200 locally advanced (stage III) pancreatic adenocarcinoma patients with irreversible electroporation: safety and efficacy. Ann Surg 2015;262:486-94; discussion 492-4. [Crossref] [PubMed]
- He S, Li S. Is irreversible electroporation (IRE) an effective and safe ablation method for local advanced pancreatic cancer: A meta-analysis. Health Sciences Review 2022;3:100029. [Crossref]
- Leen E, Picard J, Stebbing J, et al. Percutaneous irreversible electroporation with systemic treatment for locally advanced pancreatic adenocarcinoma. J Gastrointest Oncol 2018;9:275-81. [Crossref] [PubMed]
- Shen J, Pan P, Hu X, et al. Safety and Efficacy of Irreversible Electroporation in Locally Advanced Pancreatic Cancer: An Evaluation from a Surgeon’s Perspective. Cancers (Basel) 2022;14:5677. [Crossref] [PubMed]
- Sugimoto K, Moriyasu F, Tsuchiya T, et al. Irreversible Electroporation for Nonthermal Tumor Ablation in Patients with Locally Advanced Pancreatic Cancer: Initial Clinical Experience in Japan. Intern Med 2018;57:3225-31. [Crossref] [PubMed]
- He C, Wang J, Zhang Y, et al. Comparison of combination therapies in the management of locally advanced pancreatic cancer: Induction chemotherapy followed by irreversible electroporation vs radiofrequency ablation. Cancer Med 2020;9:4699-710. [Crossref] [PubMed]
- Scheffer HJ, Vroomen LG, de Jong MC, et al. Ablation of Locally Advanced Pancreatic Cancer with Percutaneous Irreversible Electroporation: Results of the Phase I/II PANFIRE Study. Radiology 2017;282:585-97. [Crossref] [PubMed]
- Ruarus AH, Vroomen LGPH, Geboers B, et al. Percutaneous Irreversible Electroporation in Locally Advanced and Recurrent Pancreatic Cancer (PANFIRE-2): A Multicenter, Prospective, Single-Arm, Phase II Study. Radiology 2020;294:212-20. [Crossref] [PubMed]
- Liu S, Qin Z, Xu J, et al. Irreversible electroporation combined with chemotherapy for unresectable pancreatic carcinoma: a prospective cohort study. Onco Targets Ther 2019;12:1341-50. [Crossref] [PubMed]
- van Veldhuisen E, Vroomen LG, Ruarus AH, et al. Value of CT-Guided Percutaneous Irreversible Electroporation Added to FOLFIRINOX Chemotherapy in Locally Advanced Pancreatic Cancer: A Post Hoc Comparison. J Vasc Interv Radiol 2020;31:1600-8. [Crossref] [PubMed]
- Geboers B, Timmer FEF, Ruarus AH, et al. Irreversible Electroporation and Nivolumab Combined with Intratumoral Administration of a Toll-Like Receptor Ligand, as a Means of In Vivo Vaccination for Metastatic Pancreatic Ductal Adenocarcinoma (PANFIRE-III). A Phase-I Study Protocol. Cancers (Basel) 2021;13:3902. [Crossref] [PubMed]
- The CROSSFIRE Study of Irreversible Electroporation for Pancreatic Cancer: Can IRE Improve Survival? HMP Global Learning Network. 2015. Available online: https://www.hmpgloballearningnetwork.com/site/iolearning/article/crossfire-study-irreversible-electroporation-pancreatic-cancer-can-ire-improve-survival
- A Randomized, Multicenter, Controlled, Unblinded Study to Assess the Safety and Efficacy of the NanoKnife® System for the Ablation of Unresectable Stage 3 Pancreatic Adenocarcinoma. 2022. Available online: https://clinicaltrials.gov/ct2/show/NCT03899636
- Zhao J, Wen X, Tian L, et al. Irreversible electroporation reverses resistance to immune checkpoint blockade in pancreatic cancer. Nat Commun 2019;10:899. [Crossref] [PubMed]
- White SB, Zhang Z, Chen J, et al. Early Immunologic Response of Irreversible Electroporation versus Cryoablation in a Rodent Model of Pancreatic Cancer. J Vasc Interv Radiol 2018;29:1764-9. [Crossref] [PubMed]
- Imran KM, Brock R, Alinezhadbalalami N, et al. IFNγ mediated PD-L1 expression mitigate the immunomodulatory effect of irreversible electroporation on pancreatic cancer J Immunol 2022;208:abstr 118.10.
- Rai ZL, Feakins R, Pallett LJ, et al. Irreversible Electroporation (IRE) in Locally Advanced Pancreatic Cancer: A Review of Current Clinical Outcomes, Mechanism of Action and Opportunities for Synergistic Therapy. J Clin Med 2021;10:1609. [Crossref] [PubMed]
- Study of the Combined Therapy of Irreversible Electroporation (IRE) and Nature Killer (NK) Cells for Advanced Pancreatic Cancer. 2019. Available online: https://clinicaltrials.gov/ct2/show/NCT02718859
- Lin M, Liang S, Wang X, et al. Short-term clinical efficacy of percutaneous irreversible electroporation combined with allogeneic natural killer cell for treating metastatic pancreatic cancer. Immunol Lett 2017;186:20-7. [Crossref] [PubMed]
- Li J, Zhang XB, Wang JJ, et al. Comparison between high-frequency irreversible electroporation and irreversible electroporation ablation of small swine liver: follow-up of DCE-MRI and pathological observations. Chin Med J (Engl) 2021;134:2081-90. [Crossref] [PubMed]
Cite this article as: Narayanan G, Jivani GG, Mahendra AM, Gentile N. A narrative review of interventional oncology approaches to treating pancreatic cancer. Dig Med Res 2024;7:12.