Formation mechanism and research progress of microenvironment before tumor metastasis: a narrative review
Introduction
Tumor metastasis is the primary cause of high mortality in malignant tumors. This is due to the invasion and widespread metastasis limiting the effectiveness of tumor treatment, thus leading to difficulty in prevention and treatment of metastatic cancers. Therefore, a complete understanding of the key components and interaction mechanisms in the process of metastasis must be acquired. From circulating tumor cells (CTCs) to formation of distal metastatic lesions, the complicated biological process of metastasis is mainly influenced by the invasiveness of tumor cells, tumor microenvironment, target organ microenvironment and other factors. In 1989, Paget et al. proposed the “seed and soil” hypothesis which states that tumor cells are capable of inducing changes in the microenvironment of distal organs through a series of mechanisms prior to the formation of metastatic lesions (1). These changes lead to a pre-metastatic niche (PMN) that supports the colonization of tumor cells for the development of metastatic lesions. In 2005, Kaplan et al. found for the first time that the bone marrow-derived VEGFR+ hematopoietic progenitor cells are involved in tumor metastasis (2). In 2013, Sceneay et al. systematically expounded the process of PMN formation (3). They proposed that tumor cells can promote the formation of PMN by reducing immune surveillance, and emphasized that PMN plays an important role in the process of metastasis. In 2016, Xuetao Cao’s team proposed six characteristics of PMN, including immunosuppression, angiogenesis and vascular permeability, lymphangiogenesis, organ affinity, inflammatory response, and structural reprogramming, and revealed the main mechanisms of PMN formation (4). In this paper, the latest progress of PMN is reviewed, and the possible clinical significance is explored to guide future research, with a primary focus to treat metastatic tumors by reversing the formation of PMNs. We present the following article in accordance with the Narrative Review reporting checklist (available at https://dx.doi.org/10.21037/dmr-21-36).
Methods
Table 1 outlines the sources of information used to compose this review. The formation of PMN is a complicated process. Active substances secreted by primary tumors, bone marrow-derived cells (BMDCs), and matrix microenvironment of distant metastatic hosts interact with each other to promote the survival, colonization and growth of tumor cells in distal target organs.
Table 1
PubMed search criteria: 1985–December 2020 |
Keywords: pre-metastatic niche; tumor metastasis; formation mechanism; research progress |
PubMed ‘related articles’ tool from identified papers |
Manual searches of the references of relevant literature |
Active substances secreted by primary tumors
Soluble factors secreted by primary tumor cells play a key role in the formation of PMN. These molecules initiate distal metastasis and determine the target organ of metastasis. It mainly includes tumor-derived secreted factors (TDSFs), extracellular vesicles (EVs), and other molecular components.
Tumor-derived secretory factor
In the initial stages of metastasis, tumor cells actively release a variety of factors via the circulatory system to act on the target organs, and the membrane receptors of the target tissue’s cells produce a response, which gradually changes its own microenvironment to be conducive for tumor development (5). Earlier studies have found that active substances such as tumor necrosis factor α (TNF-α), transforming growth factor β (TGF-β) and vascular endothelial growth factor A (VEGF-A) can induce the expression of specific inflammatory proteins in lung tissues to form a pre-metastatic microenvironment (6). Breast cancer cells can release VEGF and act on lung tissues to produce prostaglandin E2 (PGE2), inducing an inflammatory microenvironment in the lung tissue, which not only increases microangiogenesis, but also strengthens the aggregability of tumor cells, thus providing good conditions for breast cancer metastasis (7). Chemokines are also involved in the process of tumor PMN formation. Breast cancer cells secrete interleukin-6 (IL-6), which interacts with lymphatic vessel endothelial receptors in lung tissues and lymph nodes, and phosphorylates signal transducer and activator of transcription 3 (STAT3) through signal transduction. The binding of pSTAT3 and cell transcription factor upregulates the expression of chemokine ligand 5 (CCL5) which allows cancer cells into the lymphatic system, while also produces VEGF to enhance pulmonary vascular permeability and induce lymphangiosis, thus promoting tumor metastasis and colonization (8).
Additionally, it is worth noting that dietary nutrition can inhibit the formation of PMN. For example, glycyrrhizic acid can regulate lipopolysaccharide (LPS) produced by intestinal flora in weight regulation diet. Suppression of proliferation can be achieved by upregulating LPS in blood, and activating LPS in bone marrow-derived M1-like macrophages/high mobility group box 1 (HMGB1)/nuclear factor kappa-B (NF-kB) signal pathway. This in turn downregulates the secretion of inflammation-related proteins S100A8/A9, TNF-α and CCL2 by M1 macrophages to prevent the formation of tumor PMN (9).
In conclusion, TDSFs in the circulatory system can not only act on tissue cells, but also stimulate immune-related cells to mediate the formation of PMN, and non-tumor dietary nutrition can also mediate the formation of PMN.
Tumor originated extracellular vesicles
EVs mainly include exosomes and microvesicles, all of which are nano-sized membrane-structured vesicles secreted by cells, containing proteins, lipids, mRNA, miRNA, lncRNA, DNA and other active matters (10). EVs can act as a communication medium in the formation of microenvironment before metastasis. For example, tumors activate toll-like receptor-3 (TLR3) by secreting RNA-rich exosomes to induce chemokines and recruit neutrophils to the lungs to form PMN, thus promoting lung metastasis (11). Pancreatic ductal adenocarcinoma secretes a high level of tissue inhibitor of metalloprotenaises-1 (TIMP1) into circulation, which binds to the hepatic stellate cell (HSC) receptors, CD63, in the liver to activate the phosphatidylinositol 3 kinase (PI3K) pathway. The activated HSCs expresses stromal cell-derived factor 1 (SDF-1) and increases neutrophil infiltration to form PMN, which is also one of the reasons why pancreatic cancer is prone to liver metastasis (12).
EVs are closely related to immunity
Tumor-derived vesicles contain more immunosuppressive substances. Tumor-derived exosomes can cause natural killer (NK) cell dysfunction, inhibit antigen-presenting cells, block T cell activity, and increase T cell apoptosis to inhibit immunity (13). Recent studies have shown that in colorectal tumors, Dahuangzechong Pill, a traditional Chinese medicine, can inhibit the tumor-secreted CCL2 exosome to the liver, weaken the recruitment and polarization of M2 macrophages mediated by CCL2, and also reduce the expression of TGF-β, CCR2 and fibronectin in the liver to improve the hepatic fibrosis microenvironment, and in turn inhibit the formation of PMN (14). The tumor releases exocrines carrying programmed cell death protein ligand 1 (PD-L1) into the circulatory system, which bind to the programmed cell death protein 1 (PD-1) receptors on CD8+ T-cells in distant organs and inhibits its proliferation, thus reducing the ability of lymphocytes in ectopic organs to clear colonized tumor cells (15). In the metastatic sentinel lymph nodes of melanoma, its EVs are rich in proteins such as S100A8, S100A9, annexins, and intercellular adhesion molecule-1 (ICAM1), which significantly reduces the expression of CD83, CD86, Th1 polarizer FMS-related tyrosine kinase 3 ligand (Flt3L) and IL15, macrophage inflammatory protein (MIP), and inhibits the maturing of antigen-presenting dendritic cells in lung tissue that is conducive to the formation of PMN (16). However, not all exocrine bodies of the tumor are conducive for its metastasis. The exocrine secreted by premetastatic melanoma is called “non-metastatic” exosome, which highly expresses pigment epithelium-derived factor (PEDF). With the cooperation of the transcription factor nuclear receptor subfamily 4 group A member 1 (NR4A1), bone marrow-derived monocytes are recruited to form an immune line of defense, and NK cells and macrophages are also recruited to further remove tumor cells from the pre-metastatic microenvironment. Therefore, the “non-metastatic” exocrine can prevent the distant metastasis of melanoma by inducing the corresponding immune response (17).
EVs induce inflammatory response
After the EVs secreted by the tumor are absorbed by distant tissues, it induces the component cells to produce inflammatory proteins and chemotaxis inflammatory cells. This induces disorder in the metabolism and function disorder of host cells and tissue immune cells, gradually forming PMN. For example, the integrin (ITGs) in the exosome of pancreatic tumor is absorbed by Kupffer cells in the liver and induces inflammation and gradually forms PMN (18). Exosomes secreted by breast tumor cells induce PMN in lung tissues by phosphorylation of sarcoma (SRC) gene and up-regulation of S100 inflammatory gene expression (19).
EVs increase angiogenesis and vascular permeability
EVs secreted by tumors act on vascular endothelial cells in distant tissues, inducing angiogenesis and vascular leakage, and increases the metastasis and colonization of CTC in distant organs. Ovarian cancer highly expresses soluble E-cadherin, which acts on distant organs in the form of exosome bodies, activating the NF-kB pathway of endothelial cells to induce angiogenesis and increase the number of tumor cells colonized in distant tissues (20). Under the stimulation of hypoxia-inducible factor 1 (HIF1), hypoxic renal cancer cells increase the expression of carbonic anhydrase 9 (CA9), and the subsequent exosomes carrying CA9 would be absorbed by vascular endothelial cells, producing VEGF that promote angiogenesis in ectopic tissue while upregulating the expression of matrix metalloproteinase-2 (MMP2) to reshape the extracellular matrix (ECM), resulting in the formation of PMN (21). In addition, the exosome of colorectal cancer (CRC) cells is rich in MiRNA-25-3p, which down-regulates the Kruppel-like factors (KLFs) of vascular endothelial cells in liver and lung tissues that in turn increase the activity of VEGFR2 promoter, thus inducing vascular endothelial leakage and angiogenesis, and increasing colorectal hepatopulmonary metastasis. Therefore, MiRNA-25-3p exosome can be used as a biomarker of CRC metastasis in blood (22).
Tumor-derived EVs is a structure rich in protein, RNA and other active substances, which is circulated in blood to promote PMN, such as information presentation, BMDC recruitment, immune regulation, angiogenesis and so on. It involves the activation of pSTAT3, NF-kB and other signal pathways, and hypoxic environment can also participate in this process. There are also some EVs that improve the body’s immune line of defense but inhibit the formation of PMN. The presence of these EVs which are rich in active substances among blood circulation have potential application in the detection of PMN.
BMDCs
Tumor secretory substances recruit BMDCs and specific immune cells to the distal site and work with host stromal cells to reshape the microenvironment. Myeloid-derived suppressor cells (MDSCs) are a type of immature bone marrow cells, which generally appear in the early stages of PMN formation and play an important role in the microenvironment. Some studies have shown that the frequency of tumor cells captured by biosynthetic stents implanted with BMDCs is positively correlated with the frequency of CTCs in the blood, which provides reliable evidence for conformation of BMDCs involvement in the process of metastasis (23).
Chemokine
Chemokines secreted by tumor or stromal cells are the main components that affect the migration and activation of BMDCs. Recent studies have shown that CRC cells secrete VEGF-A to stimulate tumor-associated macrophages (TAMs) to produce CXCL1 in the primary foci. High concentrations of CXCL1 in the pre-metastatic liver tissue will recruit CXCR2+BMDCs to form a pre-metastatic microenvironment and promote the colonization of CRC in the liver (24). CCL12 promotes the accumulation of monocytic myeloid-derived suppressor cells (MO-MDSCs) in lung tissue. These MO-MDSCs produces IL-1β and E-selectin to promote the adhesion of tumor cells to lung endothelial cells and increase the colonization of tumor cells (25).
Integrin
Integrin is a transmembrane receptor that participates in cell-to-cell adhesion. Very late antigen-4 (VLA-4) is an integrin expressed in many BMDCs and plays an important role in cellular immunity and tumor metastasis (26). In the inflammatory microenvironment of the liver, blocking VLA-4 can reduce the tissue infiltration of MO-MDSCs by inhibiting the adhesion of endothelial cells, thus weakening the immunosuppression against liver tissue (27).
Transforming growth factor
TGF-β is one of the factors in the process of tumor progression and metastasis. In patients with non-small cell lung cancer, tumor-derived TGF-β induces CD39 and CD73 expressions in CD11b+CD33+ phenotype MDSCs by activating mechanistic target of rapamycin (mTOR)/HIF-1 signaling pathway in normal lung tissue cells, resulting in inhibition of T cell and NK cell activity (28). In lung tissue, the production of TGF-β by CCR2+MO-MDSCs promotes pulmonary fibroblasts to release tissue inhibitor of metalloproteinase-1 (TIMP1), which forms a non-degradable collagen microenvironment, leading to pulmonary fibrosis (29).
Vascular growth factor
The vascular systems in distal metastasis are often malformed and highly permeable, which is conducive to tumor cell adhesion and infiltration, which is all due to the mediation of VEGF. Early studies have shown that tumor-derived VEGF can mobilize VEGFR1+ hematopoietic progenitor cells from bone marrow into distant organs. These BMDCs express fibronectin receptor integrin and promote fibroblasts to produce fibronectin and MMP9, gradually forming favorable conditions for tumor spread and colonization. However, VEGF also has the function of recruiting BMDCs. In lung tissue, tumor can induce the overexpression of cytochrome P450 in TAMs. P450 activates the STAT3 signal pathway of TAMs to promote the polarization of M2 macrophages. M2 macrophages secrete VEGF and TGF-β to recruit VEGFR1+BMDCs and fibroblasts, further inducing the formation of PMN (30).
Extracellular vesicles
The exosome secreted by the tumor contributes to the recruitment of MDSCs. After ingesting exosomes rich in macrophage migration inhibitory factor (MIF) secreted by pancreatic cancer cells, Kupffer cells in the liver produce TGF-β, and HSCs upregulate the expression of fibronectins. This fibrotic microenvironment increases the recruitment of bone marrow-derived macrophages, resulting in increased liver metastasis of pancreatic cancer (31). At the same time, MDSCs can also secrete exosomes to promote tumor progression. It has been found that in the mouse model of breast cancer, the exosomes secreted by MDSCs are rich in inflammatory protein S100A8/A9, which promotes macrophage differentiation to M2 type, which is beneficial to tumor progression (32). Adriamycin-treated breast cancer cells initiate IL-13R+MDSCs recruitment, and these MDSCs secrete miR-126a exosomes to promote IL-13+Th2 cell proliferation and tumor angiogenesis, thus increasing tumor lung metastasis (33).
Vitamins
Vitamins can mediate the biological activity of MDSCs. Early studies have found that in patients with squamous cell carcinoma of the head and neck, vitamin D3 treatment can reduce the level of bone marrow-derived CD34+ progenitor cells involved in immunosuppression within the tumor, but can increase the chemotaxis of mature dendritic cells, delaying the time of tumor recurrence (34). In clinical trials, patients with renal cell carcinomas with high serum levels of all-trans retinoic acid (ATRA) are detected to have fewer MDSCs and more powerful T cells compared with the normal group, which may be related to the fact that ATRA helps to directly distinguish the premise of antigen presentation and increases the efficiency of immune cells (35). Some new studies have shown that vitamin D can interfere with the induction of MDSCs by miR-155 exocrine secreted by lymphocytic leukocytes (36). In the lipopolysaccharide-immunosuppressed mice model, ATRA reduces the formation of MDSCs and enhances immune function by inhibiting the proliferation of CD34+ premise cells (37).
The formation of PMN is often mediated by tumor-derived BMDCs. Primary tumor cells induce immature cells in bone marrow, such as MDSCs, VEGFR1+ hematopoietic progenitors and neutrophils, as well as various phenotypes of BMDCs, such as IL-13R+, CXCR2+, CD11b+CD33+ and so on. These BMDCs are recruited to target organs to secrete active substances and interact with host stromal cells to coordinate the formation of PMN. In addition, vitamins can regulate the biological activity of BMDCs. Blocking the biological activity of BMDCs related to PMN can be used as a new strategy for the prevention and treatment of metastasis.
Stromal cells and their components in host tissue
The ECM environment of the host is mainly composed of fibroblasts, endothelial cells, immune cells, ECM, vascular and lymphoid duct system. Tumor acts on the host through cytokines, extracellular vesicles and recruitment of bone-derived cells. The purpose is to alter or even reconstruct the matrix environment to facilitate metastasis and colonization. For example, tumor cells activate PI3K/protein kinase B (AKt) signal pathway in fibroblasts in PMN, which promotes the production of SDF-1, TGF-β, MMP and fibronectin, thus remodeling the ECM (38). HSCs are affected by hepatocyte steatosis and secrete large amounts of IL-1, VEGF and TGF-β to induce the formation of pre-metastatic microenvironment (39).
Nutrition and metabolism
Tumor cells can also change the nutritional composition and energy metabolism of ECM. Recent studies have reported that breast cancer cells absorb and breakdown proline in the lung tissue into energy supply to increase the success rate of lung metastasis (40). In addition, breast cancer cells rely on pyruvate in the ECM to increase collagen hydroxylation for microenvironment remodeling in the lung metastatic microenvironment (41). Tumor-derived RNA can mediate the metabolism of lung and brain cells. miR-122 downregulates pyruvate kinase in normal cells and inhibits their glucose uptake, increases ECM nutrients and redistributes energy metabolism, which can promote tumor metastasis (42).
Biological scaffold
In terms of microenvironment matrix, acellular scaffold is a scaffolding material engineered with decellularization technology, which can be used as substrate and 3D base of cell culture, and can simulate the composition of matrix and structure of microenvironment for scientific research (43). For example, when isolating the normal lung matrix of mice with decellularization technology, it was observed that metastatic breast cancer cells could grow in healthy lung acellular scaffolds, while only a small number of non-metastatic breast cancer cells survived. In the experiment, by knocking out zinc finger E-box binding protein (ZEB) gene, tumor colonization could then be inhibited, thus showing that biological scaffolds can be used as a reliable tool for the study of host ECM (44). In the mice breast cancer model, the three-dimensional acellular scaffolds simulating metastatic liver and lung tissues ECM were compared and analyzed proteomically with those simulating healthy liver and lung tissues. It was found that myeloperoxidase (MPO) in ECM mediates tumor cell colonization. It was then suggested that MPO could be a signal protein of breast cancer PMN (19). Additionally, the scaffold containing human osteoblasts was used to simulate the environment of matrix mineralization in human bone. Under electron microscope, the ECM simulated by the scaffold was not only very similar to that of human bone matrix, but also found that the cancer cells had increased adhesion to bone fibers when mediated by integrin, leading to phosphorylation of focal adhesion kinase (FAK) and extracellular regulated kinase 1/2 (ERK1/2), and finally upregulating the expression of MMPs, forming a favorable environment for cancer cell metastasis (45).
Host stromal cells and its components directly affect the formation of PMN. Under the influence of related cells and active substances, host stromal cells and mobilized BMDCs produce responses, like inducing tissue fibrosis, inflammation and matrix remodeling, and also change tissue nutritional composition and metabolic rearrangement, which provides favorable conditions for PMN. Monitoring the changes of specific components in the ECM can evaluate the degree of PMN formation and allow for timely diagnosis and treatment before metastasis occurs (Figure 1).
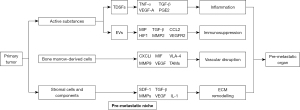
Summary
Tumor metastasis is closely related to the formation of PMN. By expounding and analyzing literatures in recent years, this paper summarizes the important role and mechanism of active substances, related cells and EVs in the formation of PMN, and once again reveals the complexity of studying biological activities pertaining to metastasis, and the interactions between various factors promoting the formation of PMN. At present, there are still some questions about the research on PMN mechanism, including: (I) How does tumor microenvironment affect the formation of PMN? (II) If TDSFs and EVs in the circulatory system are suppressed, can PMN still form? Can the primary tumor activate other pathways to induce PMN? (III) Can EVs and MDSCs be used as reliable markers for PMN formation? How can we accurately evaluate the degree of PMN formation? (IV) Although the primary tumor induces PMN formation through various ways, can it directly alter the ECM in distant ectopic tissue to reverse PMN formation? (V) What role do the many kinds of nutritional compositions and energy metabolisms in the body play in the formation of PMN respectively? What are their specific mechanisms? Can the nutritional composition and energy metabolism of tissue cells become a new scheme that affects the formation of PMN? From all the above, it is necessary for future researches to develop models and related technologies that closely simulates the internal environment of the human body and create more targeted experimental design to answer these questions.
With the development of material sciences and tissue engineering technology, new tools such as acellular biological scaffolds with good biocompatibility, magnetic nanoparticles and single photon emission computed tomography (SPECT) allow for the studying of the formation and mechanism of PMN. The aim is to screen out biomarkers from circulatory system or ECM for detection and evaluation of PMN, and pinpoint disruption of key components to effectively prevent and treat PMN. Better understanding of the formation mechanism of PMN will lead to clinical transformation, and eventually provide a better plan for the prevention and treatment of tumor metastasis.
Acknowledgments
Funding: The Science and Technology Planning Project of Shenzhen, China (JCYJ20190809142807444).
Footnote
Reporting Checklist: The authors have completed the Narrative Review reporting checklist. Available at https://dx.doi.org/10.21037/dmr-21-36
Conflicts of Interest: All authors have completed the ICMJE uniform disclosure form (available at https://dx.doi.org/10.21037/dmr-21-36). The authors have no conflicts of interest to declare.
Ethical Statement: The authors are accountable for all aspects of the work in ensuring that questions related to the accuracy or integrity of any part of the work are appropriately investigated and resolved.
Open Access Statement: This is an Open Access article distributed in accordance with the Creative Commons Attribution-NonCommercial-NoDerivs 4.0 International License (CC BY-NC-ND 4.0), which permits the non-commercial replication and distribution of the article with the strict proviso that no changes or edits are made and the original work is properly cited (including links to both the formal publication through the relevant DOI and the license). See: https://creativecommons.org/licenses/by-nc-nd/4.0/.
References
- Paget S. The distribution of secondary growths in cancer of the breast. 1889. Cancer Metastasis Rev 1989;8:98-101. [PubMed]
- Kaplan RN, Riba RD, Zacharoulis S, et al. VEGFR1-positive haematopoietic bone marrow progenitors initiate the pre-metastatic niche. Nature 2005;438:820-7. [Crossref] [PubMed]
- Sceneay J, Smyth MJ, Möller A. The pre-metastatic niche: finding common ground. Cancer Metastasis Rev 2013;32:449-64. [Crossref] [PubMed]
- Liu Y, Cao X. Characteristics and Significance of the Pre-metastatic Niche. Cancer Cell 2016;30:668-81. [Crossref] [PubMed]
- Peinado H, Lavotshkin S, Lyden D. The secreted factors responsible for pre-metastatic niche formation: old sayings and new thoughts. Semin Cancer Biol 2011;21:139-46. [Crossref] [PubMed]
- Hiratsuka S, Watanabe A, Sakurai Y, et al. The S100A8-serum amyloid A3-TLR4 paracrine cascade establishes a pre-metastatic phase. Nat Cell Biol 2008;10:1349-55. [Crossref] [PubMed]
- Liu S, Jiang M, Zhao Q, et al. Vascular endothelial growth factor plays a critical role in the formation of the pre-metastatic niche via prostaglandin E2. Oncol Rep 2014;32:2477-84. [Crossref] [PubMed]
- Lee E, Fertig EJ, Jin K, et al. Breast cancer cells condition lymphatic endothelial cells within pre-metastatic niches to promote metastasis. Nat Commun 2014;5:4715. [Crossref] [PubMed]
- Qiu M, Huang K, Liu Y, et al. Modulation of intestinal microbiota by glycyrrhizic acid prevents high-fat diet-enhanced pre-metastatic niche formation and metastasis. Mucosal Immunol 2019;12:945-57. [Crossref] [PubMed]
- van Niel G, D'Angelo G, Raposo G. Shedding light on the cell biology of extracellular vesicles. Nat Rev Mol Cell Biol 2018;19:213-28. [Crossref] [PubMed]
- Liu Y, Gu Y, Han Y, et al. Tumor Exosomal RNAs Promote Lung Pre-metastatic Niche Formation by Activating Alveolar Epithelial TLR3 to Recruit Neutrophils. Cancer Cell 2016;30:243-56. [Crossref] [PubMed]
- Grünwald B, Harant V, Schaten S, et al. Pancreatic Premalignant Lesions Secrete Tissue Inhibitor of Metalloproteinases-1, Which Activates Hepatic Stellate Cells Via CD63 Signaling to Create a Premetastatic Niche in the Liver. Gastroenterology 2016;151:1011-1024.e7. [Crossref] [PubMed]
- Ludwig S, Floros T, Theodoraki MN, et al. Suppression of Lymphocyte Functions by Plasma Exosomes Correlates with Disease Activity in Patients with Head and Neck Cancer. Clin Cancer Res 2017;23:4843-54. [Crossref] [PubMed]
- Chen C, Yao X, Xu Y, et al. Dahuang Zhechong Pill suppresses colorectal cancer liver metastasis via ameliorating exosomal CCL2 primed pre-metastatic niche. J Ethnopharmacol 2019;238:111878. [Crossref] [PubMed]
- Chen G, Huang AC, Zhang W, et al. Exosomal PD-L1 contributes to immunosuppression and is associated with anti-PD-1 response. Nature 2018;560:382-6. [Crossref] [PubMed]
- Maus RLG, Jakub JW, Nevala WK, et al. Human Melanoma-Derived Extracellular Vesicles Regulate Dendritic Cell Maturation. Front Immunol 2017;8:358. [Crossref] [PubMed]
- Plebanek MP, Angeloni NL, Vinokour E, et al. Pre-metastatic cancer exosomes induce immune surveillance by patrolling monocytes at the metastatic niche. Nat Commun 2017;8:1319. [Crossref] [PubMed]
- Hoshino A, Costa-Silva B, Shen TL, et al. Tumour exosome integrins determine organotropic metastasis. Nature 2015;527:329-35. [Crossref] [PubMed]
- Aguado BA, Caffe JR, Nanavati D, et al. Extracellular matrix mediators of metastatic cell colonization characterized using scaffold mimics of the pre-metastatic niche. Acta Biomater 2016;33:13-24. [Crossref] [PubMed]
- Tang MKS, Yue PYK, Ip PP, et al. Soluble E-cadherin promotes tumor angiogenesis and localizes to exosome surface. Nat Commun 2018;9:2270. [Crossref] [PubMed]
- Horie K, Kawakami K, Fujita Y, et al. Exosomes expressing carbonic anhydrase 9 promote angiogenesis. Biochem Biophys Res Commun 2017;492:356-61. [Crossref] [PubMed]
- Zeng Z, Li Y, Pan Y, et al. Cancer-derived exosomal miR-25-3p promotes pre-metastatic niche formation by inducing vascular permeability and angiogenesis. Nat Commun 2018;9:5395. [Crossref] [PubMed]
- Bersani F, Lee J, Yu M, et al. Bioengineered implantable scaffolds as a tool to study stromal-derived factors in metastatic cancer models. Cancer Res 2014;74:7229-38. [Crossref] [PubMed]
- Wang D, Sun H, Wei J, et al. CXCL1 Is Critical for Premetastatic Niche Formation and Metastasis in Colorectal Cancer. Cancer Res 2017;77:3655-65. [Crossref] [PubMed]
- Shi H, Zhang J, Han X, et al. Recruited monocytic myeloid-derived suppressor cells promote the arrest of tumor cells in the premetastatic niche through an IL-1β-mediated increase in E-selectin expression. Int J Cancer 2017;140:1370-83. [Crossref] [PubMed]
- Schlesinger M, Bendas G. Contribution of very late antigen-4 (VLA-4) integrin to cancer progression and metastasis. Cancer Metastasis Rev 2015;34:575-91. [Crossref] [PubMed]
- Resheq YJ, Menzner AK, Bosch J, et al. Impaired Transmigration of Myeloid-Derived Suppressor Cells across Human Sinusoidal Endothelium Is Associated with Decreased Expression of CD13. J Immunol 2017;199:1672-81. [Crossref] [PubMed]
- Li J, Wang L, Chen X, et al. CD39/CD73 upregulation on myeloid-derived suppressor cells via TGF-β-mTOR-HIF-1 signaling in patients with non-small cell lung cancer. Oncoimmunology 2017;6:e1320011. [Crossref] [PubMed]
- Lebrun A, Lo Re S, Chantry M, et al. CCR2+ monocytic myeloid-derived suppressor cells (M-MDSCs) inhibit collagen degradation and promote lung fibrosis by producing transforming growth factor-β1. J Pathol 2017;243:320-30. [Crossref] [PubMed]
- Chen XW, Yu TJ, Zhang J, et al. CYP4A in tumor-associated macrophages promotes pre-metastatic niche formation and metastasis. Oncogene 2017;36:5045-57. [Crossref] [PubMed]
- Costa-Silva B, Aiello NM, Ocean AJ, et al. Pancreatic cancer exosomes initiate pre-metastatic niche formation in the liver. Nat Cell Biol 2015;17:816-26. [Crossref] [PubMed]
- Burke M, Choksawangkarn W, Edwards N, et al. Exosomes from myeloid-derived suppressor cells carry biologically active proteins. J Proteome Res 2014;13:836-43. [Crossref] [PubMed]
- Deng Z, Rong Y, Teng Y, et al. Exosomes miR-126a released from MDSC induced by DOX treatment promotes lung metastasis. Oncogene 2017;36:639-51. [Crossref] [PubMed]
- Walsh JE, Clark AM, Day TA, et al. Use of alpha,25-dihydroxyvitamin D3 treatment to stimulate immune infiltration into head and neck squamous cell carcinoma. Hum Immunol 2010;71:659-65. [Crossref] [PubMed]
- Kusmartsev S, Su Z, Heiser A, et al. Reversal of myeloid cell-mediated immunosuppression in patients with metastatic renal cell carcinoma. Clin Cancer Res 2008;14:8270-8. [Crossref] [PubMed]
- Bruns H, Böttcher M, Qorraj M, et al. CLL-cell-mediated MDSC induction by exosomal miR-155 transfer is disrupted by vitamin D. Leukemia 2017;31:985-8. [Crossref] [PubMed]
- Martire-Greco D, Rodriguez-Rodrigues N, Castillo LA, et al. Novel Use of All-Trans-Retinoic Acid in A Model of Lipopolysaccharide-Immunosuppression to Decrease the Generation of Myeloid-Derived Suppressor Cells by Reducing the Proliferation of CD34+ Precursor Cells. Shock 2017;48:94-103. [Crossref] [PubMed]
- Yamamura Y, Asai N, Enomoto A, et al. Akt-Girdin signaling in cancer-associated fibroblasts contributes to tumor progression. Cancer Res 2015;75:813-23. [Crossref] [PubMed]
- Mikuriya Y, Tashiro H, Kuroda S, et al. Fatty liver creates a pro-metastatic microenvironment for hepatocellular carcinoma through activation of hepatic stellate cells. Int J Cancer 2015;136:E3-13. [Crossref] [PubMed]
- Elia I, Broekaert D, Christen S, et al. Proline metabolism supports metastasis formation and could be inhibited to selectively target metastasizing cancer cells. Nat Commun 2017;8:15267. [Crossref] [PubMed]
- Elia I, Rossi M, Stegen S, et al. Breast cancer cells rely on environmental pyruvate to shape the metastatic niche. Nature 2019;568:117-21. [Crossref] [PubMed]
- Fong MY, Zhou W, Liu L, et al. Breast-cancer-secreted miR-122 reprograms glucose metabolism in premetastatic niche to promote metastasis. Nat Cell Biol 2015;17:183-94. [Crossref] [PubMed]
- Crapo PM, Gilbert TW, Badylak SF. An overview of tissue and whole organ decellularization processes. Biomaterials 2011;32:3233-43. [Crossref] [PubMed]
- Xiong G, Flynn TJ, Chen J, et al. Development of an ex vivo breast cancer lung colonization model utilizing a decellularized lung matrix. Integr Biol (Camb) 2015;7:1518-25. [Crossref] [PubMed]
- Taubenberger AV, Quent VM, Thibaudeau L, et al. Delineating breast cancer cell interactions with engineered bone microenvironments. J Bone Miner Res 2013;28:1399-411. [Crossref] [PubMed]
Cite this article as: Chen J, Liu S, Jiang J, Kuo Z, Li J, Hong C, Yu H, Li M, Wu W, Ma C. Formation mechanism and research progress of microenvironment before tumor metastasis: a narrative review. Dig Med Res 2021;4:70.